Advanced Insights into Systems Control Realm
In the ever – evolving landscape of technology and engineering, systems control stands as a cornerstone concept. Systems control, in its essence, refers to the ability to manage and manipulate the behavior of a system to achieve desired outcomes. Whether it’s a simple mechanical system, a complex industrial process, or a sophisticated software – based system, the principles of systems control play a crucial role.
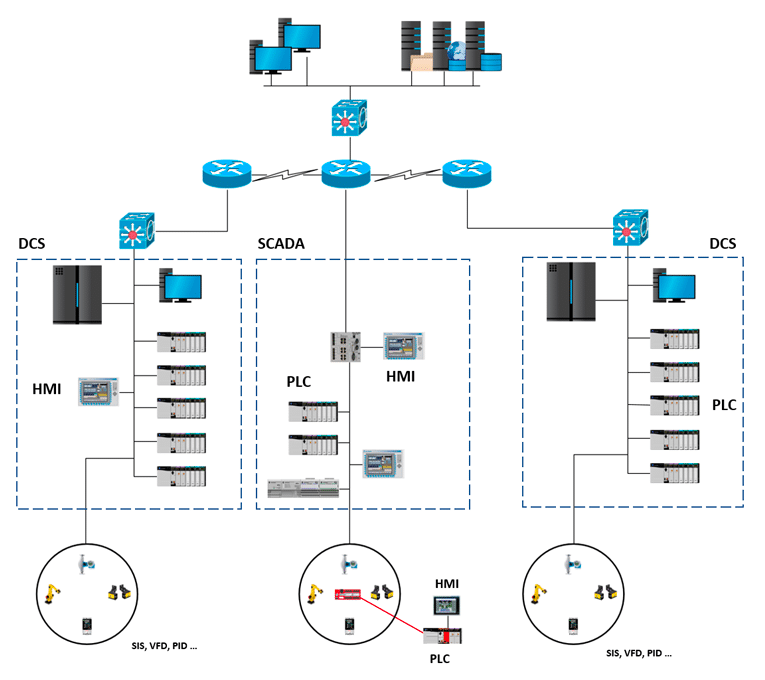
The Basics of Systems Control
At its most fundamental level, systems control involves three key elements: the system itself, the controller, and the feedback loop. The system is the entity that we want to control. For example, in a temperature – controlled room, the room is the system. The controller is the device or algorithm that makes decisions to adjust the system. In the case of the temperature – controlled room, the thermostat is the controller. The feedback loop is what allows the controller to receive information about the system’s current state. A temperature sensor in the room provides feedback to the thermostat.
Types of Systems Control
There are two main types of systems control: open – loop control and closed – loop control.
- Open – loop control: In an open – loop control system, the controller sends signals to the system without considering the actual output of the system. For instance, a simple timer – based lawn sprinkler system is an open – loop system. The timer turns the sprinklers on for a set amount of time, regardless of whether the lawn is actually getting enough water or not. 🌱
- Closed – loop control: Also known as feedback control, a closed – loop system continuously monitors the output of the system and adjusts the input based on the feedback. A cruise control system in a car is a classic example. The system measures the speed of the car (output), and if the speed deviates from the set value, the controller adjusts the throttle (input) to maintain the desired speed. 🚗
Type of Control
|
Definition
|
Example
|
Open – loop control
|
Controller acts without considering system output
|
Timer – based lawn sprinkler
|
Closed – loop control
|
Controller adjusts input based on system output feedback
|
Cruise control in a car
|
Applications of Systems Control
Industrial Automation
In industrial settings, systems control is used extensively in automation processes. Robots on an assembly line are controlled to perform specific tasks with high precision. The control system ensures that each robot moves to the correct position, picks up the right parts, and assembles them accurately. For example, in a car manufacturing plant, robots are programmed to weld parts together. The control system monitors the position of the welding torch and the parts being welded, making real – time adjustments to ensure a strong and precise weld. 🏭
Aerospace Engineering
In the aerospace industry, systems control is vital for the safe and efficient operation of aircraft and spacecraft. Flight control systems use a combination of sensors, actuators, and control algorithms to maintain the stability and trajectory of an aircraft. For example, the autopilot system in an airplane uses information from sensors such as gyroscopes and accelerometers to adjust the ailerons, rudder, and elevator to keep the plane on the desired course. 🛩️
Industry
|
Application of Systems Control
|
Benefits
|
Industrial Automation
|
Robot control in assembly lines
|
Higher precision, increased productivity
|
Aerospace Engineering
|
Flight control systems
|
Improved safety, fuel efficiency
|
Smart Grids
In the context of energy management, smart grids rely on systems control to optimize the generation, distribution, and consumption of electricity. Sensors are placed throughout the grid to monitor the power flow, voltage levels, and load demands. Based on this information, the control system can adjust the output of power plants, manage energy storage systems, and even regulate the electricity consumption of large industrial customers. This helps in reducing energy waste, improving grid stability, and integrating renewable energy sources more effectively. ⚡
Key Concepts in Systems Control
Transfer Functions
A transfer function is a mathematical representation of the relationship between the input and output of a linear time – invariant system. It is used to analyze the behavior of a system in the frequency domain. For example, in an electrical circuit, the transfer function can describe how the voltage across a capacitor responds to an input voltage signal. By analyzing the transfer function, engineers can determine the system’s stability, bandwidth, and other important characteristics.
State – Space Representation
State – space representation is another way to model a system, especially useful for non – linear and multi – variable systems. It represents the system in terms of its internal state variables, inputs, and outputs. In a robotic system, the state variables could include the position and velocity of each joint. The state – space equations describe how these state variables change over time in response to the inputs (such as control signals) and the system’s dynamics.
Stability Analysis
Stability is a crucial aspect of systems control. A stable system is one that, when perturbed from its equilibrium state, will return to that state over time. There are various methods for analyzing the stability of a system, such as the Routh – Hurwitz criterion, Nyquist stability criterion, and Lyapunov stability theory. For example, in a chemical process control system, ensuring stability is essential to prevent runaway reactions or other dangerous situations.
Challenges in Systems Control
Non – linearity
Many real – world systems are non – linear, which means that the relationship between the input and output is not a simple linear one. Non – linear systems are much more difficult to analyze and control compared to linear systems. For example, the aerodynamic forces acting on an aircraft change non – linearly with the speed, altitude, and angle of attack. Developing control algorithms for such non – linear systems requires advanced mathematical techniques and often involves approximation methods.
Uncertainty
Systems control also has to deal with uncertainty. There could be uncertainties in the system parameters, such as the mass of a moving object in a mechanical system, or in the external disturbances acting on the system. For example, in a wind – turbine control system, the wind speed and direction are highly variable and uncertain. Uncertainty can make it challenging to design a controller that can perform well under all possible conditions.
Complexity
As systems become more complex, with multiple interacting components and subsystems, the task of systems control becomes increasingly difficult. For example, in a modern smart city infrastructure, there are numerous systems such as traffic control, water supply, and power distribution that interact with each other. Coordinating the control of these systems to achieve overall efficiency and sustainability is a complex challenge.
Future Trends in Systems Control
Integration with Artificial Intelligence
The integration of artificial intelligence (AI) and machine learning (ML) techniques into systems control is a promising trend. AI – based controllers can learn from the data collected by the system and adapt their control strategies in real – time. For example, in autonomous vehicles, machine – learning algorithms can analyze the sensor data (such as camera images and LiDAR scans) to make driving decisions, such as when to accelerate, brake, or turn. 🚙
Distributed Control Systems
With the growth of the Internet of Things (IoT), distributed control systems are becoming more prevalent. In a distributed control system, multiple controllers are networked together to control a large – scale system. For example, in a large – scale industrial facility, different controllers at various locations can communicate with each other to optimize the overall process. This allows for more flexibility, scalability, and reliability in systems control. 🌐
Green and Sustainable Control
As the world focuses more on environmental sustainability, systems control is also moving towards greener solutions. In energy – related systems, such as power plants and building energy management systems, control strategies are being developed to minimize energy consumption and reduce emissions. For example, in a building, the control system can optimize the operation of heating, ventilation, and air – conditioning (HVAC) systems based on the occupancy, weather conditions, and energy prices. 🏢
Systems control is a vast and dynamic field that has far – reaching applications in various industries. From industrial automation to aerospace engineering and smart grids, the principles of systems control are essential for ensuring the efficient, safe, and reliable operation of systems. Despite the challenges posed by non – linearity, uncertainty, and complexity, ongoing research and technological advancements, such as the integration of AI and the development of distributed control systems, are opening up new possibilities for the future of systems control. As we continue to explore and innovate in this field, systems control will undoubtedly play an even more significant role in shaping our technological landscape and improving our quality of life. 🌟